Written by: Talia McDaniel
The pH scale is simultaneously one of the most ubiquitous ideas in chemistry and one of the least explained. Almost anyone involved in the world of STEM has a basic understanding (no pun intended) of acids, bases, and buffers, but an understanding of how these concepts work is far from common knowledge. Today, we’ll take a deep dive into the pH scale—how it came to be, how it works, and how it applies to life in fields like medicine and agriculture.
Though the idea of compounds being “acidic” existed in simple forms as early as the era of the ancient Greeks, it wasn’t until the 1700s that scientific interest in understanding the nature of acids and bases truly began. Researchers began their inquiry by experimenting to determine what substances, if any, formed the underlying properties of acids. Initially, it was thought that oxygen could be responsible; however, not all acids contain oxygen. As later researchers eventually discovered, the only element all acids have in common is hydrogen—specifically, positively charged hydrogen ions (H+), which they release in one way or another when added to water. Bases were originally understood as compounds that neutralized acids, but, later on, the definition was narrowed down: bases are substances that release an abundance of hydroxide ions (OH-) when added to water.
With a rudimentary understanding of acids and bases established, all that remained was to develop a system that could smoothly categorize them. This was accomplished in 1909 by the Danish chemist Søren Peder Lauritz Sørenson. Sørenson designed a simple logarithmic formula that quantified the concentration of hydrogen ions in a solution as follows:
pH = -log_10[H+]
Where [H+] is replaced by the number of hydrogen ions in the solution, represented in molar form.
As you might have guessed from the presence of a value “pH,” this equation gave way to the pH scale: the fundamental measurement we use to determine how acidic or alkaline (basic) a substance is. The scale anchors at seven, which is a completely neutral pH—the pH value of pure water. Solutions that have a higher concentration of hydrogen ions than pure water (acids) result in a lower output value from the pH formula; therefore, any pH value below seven is acidic. pH values above seven are alkaline, because their lower concentration of hydrogen ions results in a higher output value from the formula. The pH scale goes from zero to fourteen, and it is commonly represented in visual form like this:
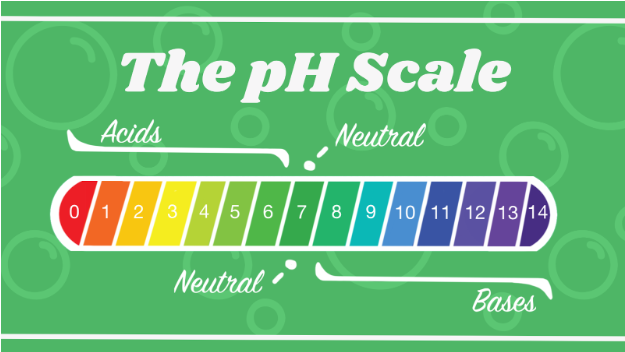
So now that we’ve uncovered the basics, how do acids, bases, and buffers work on a chemical level?
In order to understand this, we must look to pure water, the substance on which the pH scale is balanced. As you almost certainly know, water molecules are made of two hydrogen atoms and one oxygen atom—H2O. These two basic parts each carry their own charges, with the oxygen atom having a greater electronegative charge than the hydrogen atoms. Because of this difference in charges, electrons are drawn towards the oxygen atom and repelled from the hydrogen atoms. This makes water molecules polar, which is crucial for many of water’s functions in our world. Most importantly for our discussion, however, this polarity also facilitates the dissociation of water into two ions: H+ (in the form of H3O+) and OH-. About two water molecules in every billion will, at any given moment, lose an electron to another water molecule, producing the charged ions H3O+ and OH-. This change is reversible, and the two ions can easily recombine to form ordinary H2O. At any given moment, a room temperature sample of water contains approximately 1 × 10^-7 hydrogen ions per Mole that have been “liberated” from their original water molecule form. If you recall the equation for a solution’s pH, pH = -log_10[H+], you might be able to see from this number exactly why water has a pH of seven.
Because the separated ions in water are easily able to reunite, water’s electrical and chemical properties are very neutral. When other substances are added to it, however, that can all change. Our operational definitions for acids and bases rely on what happens when we add a substance to water. If the substance reacts with the water molecules in such a way that the concentration of hydrogen ions in the solution increases, we call it an acid. If the substance reacts with the water molecules in a way that captures hydrogen ions (thus increasing the concentration of hydroxide ions in the solution), we call it a base. Let’s look at two examples that demonstrate this concept.
First, let’s imagine that we dissolved some carbon dioxide into a sample of pure water. When carbon dioxide diffuses into water, some of the carbon dioxide molecules will react with the water molecules to form carbonic acid:

CO2 + H2O <> H2CO3
As the arrows indicate, this reaction is reversible, so the carbonic acid can return back to being carbon dioxide and water. Because the carbonic acid exists in a solution full of polar water molecules, however, it is more quick to dissociate into ions, instead. In particular, a hydrogen ion and a bicarbonate ion are released:

H2CO3 <> HCO3- + H+
This reaction is also reversible, so the hydrogen ions can be reclaimed, but the overall effect of adding carbon dioxide to water is to increase the hydrogen ion concentration of the solution. This is why we consider carbonic acid to be an acid!
On the other end of the scale, let’s consider what happens when we add a base, like ammonia (NH3), to water. When ammonia is added to water, it forms ammonium hydroxide:

NH3 + H2O <> NH4OH
Much like with the reaction between carbon dioxide and water, the product in this reaction, ammonium hydroxide, dissociates into ions when placed in water:

NH4OH <> NH4+ + OH-
Crucially, when it dissociates, it releases a hydroxide ion. Hydroxide ions very readily combine with any hydrogen ions present to form water; this means that they reduce the hydrogen ion concentration of the solution. This is why we consider ammonia to be a base.
Carbon dioxide/carbonic acid and ammonia are very weak examples of acids and bases, but, on the chemical level, any acid or base goes through very similar processes when added to water. The way they react with water results either in a dissociation full of hydrogen ions or a dissociation full of hydroxide ions. Hydrogen ions by themselves are the basis for acidification, and hydroxide ions “sponge up” loose hydrogen ions to make their solutions more basic.
One final element worth considering in the grand scheme of acids and bases in chemistry is buffers. Buffers are pairs of weak acids or weak bases and their conjugates, or the bases/acids that “match” them in such a way that they exist in equilibrium. When other acids or bases get added to the solution, the existence of acids and bases in the buffer is able to neutralize them. This stabilizing property only extends until either the acid or base in the buffer is depleted; after that point, the pH changes sharply because both the conjugate and the added material are now present, unchecked, in the solution.
Though it’s easy to visualize acids and bases primarily in the setting of a chemistry lab, they are found all throughout the world around us. The way acids and bases interact have a myriad of impacts on our day-to-day lives, and we’ll explore a few of those applications here.
Medicine — The Delicate Balance of Our Blood
Like any aqueous solution, blood has a known pH. As a general rule, the pH of blood exists in a very narrow range between 7.35 and 7.45. Various buffering systems exist in our body to maintain this ideal range. Our cardiopulmonary systems, for example, help manage the acidity of our blood by increasing or decreasing the rate at which carbon dioxide is expelled. Carbon dioxide is a byproduct of cellular respiration, and, at any moment, there is a lot of it circulating in our bloodstreams. As mentioned earlier, carbon dioxide reacts with water to form carbonic acid, so its presence in our water-based blood has a slight acidifying effect. This isn’t a bad thing; in fact, carbon dioxide is a crucial part of our body’s buffer system. When something else makes blood too acidic, however, the body can compensate by expelling more carbon dioxide than usual. Carbon dioxide is naturally expelled when we breathe, so an increase in our rate of respiration (combined with an increased heart rate to bring as much carbon dioxide to the lungs as possible) can help free up the bases present in our bodies to address the increased presence of acid.
Disorders of the blood pH can have serious consequences, and, when our bodies’ natural buffering systems fail, medical experts must harness the properties of acids and bases to address the imbalance. Failing to do so can have severe effects on the body, including coma and death, so taking action is crucial.
In the medical field, disorders of the blood pH are sorted into four different categories: metabolic alkalosis, metabolic acidosis, respiratory alkalosis, and respiratory acidosis. The respiratory versions occur when a person, for any reason, breathes too much or too little. Breathing too much, as mentioned earlier, results in a great deal of carbon dioxide being expelled. If there isn’t an excess of acid present in the body, an increased rate of breathing can tip the blood’s balance towards being too basic. Breathing too little, on the other hand, has the opposite effect; not enough carbon dioxide is expelled, so the blood becomes more acidic.
While respiratory alkalosis and acidosis can certainly cause problems or be a sign of more serious issues, medical professionals are typically more concerned with metabolic acidosis and alkalosis. These are conditions where the balance is thrown off by the addition of something to the body’s system that either acidifies or alkalizes the blood. These sorts of changes are often more drastic, leading to more dangerous side-effects. Causes for metabolic acidosis and alkalosis can be external (i.e. overdosing on certain medications like antacids or laxatives) or internal (i.e. medical conditions like diabetes or dehydration). In order to appropriately treat metabolic pH imbalances, doctors must be able to determine the root cause and address it appropriately—though adding weak acids or bases to the blood intravenously can help stabilize the situation in the interim.
Agriculture and Horticulture — pH Sensitive Plants
An understanding of pH is also an important part of plant husbandry. Plants require very particular environmental conditions to grow, and the presence of too much acid or base in their surroundings can throw off their ideal balance. High soil acidity, in particular, tends to be a more common issue, and it can have a devastating effect on agriculture if left unchecked.
Most plants thrive in slightly acidic conditions, in soil with a pH range of five to six. If the soil becomes more acidic than that, however, vital nutrients like nitrates, potassium, and magnesium are leached away as they react with the prevalent hydrogen ions. The acidic conditions also make metals like aluminum, iron, and zinc more available, which is toxic to many plants.
High soil acidity can be a byproduct of how land is managed for agricultural use. Too much of certain fertilizers, a lack of natural plant and animal wastes, or an overabundance of decaying plant material can have an acidifying effect on the soil. Soil acidity can also simply result from the geology of the surrounding land and local water conditions. In either case, farmers who want their crop to thrive must remedy the imbalance by neutralizing the acid with some sort of base. Lime—a strong base containing calcium oxides and hydroxides that is derived from rocks like limestone—is the traditional remedy.
While a plant’s ability to thrive is the primary application of pH, the way some plants look is also impacted by pH. Certain species of hydrangea, for example, will change the color of their flowers according to the pH of their soil—a natural litmus test! In standard, slightly acidic, conditions, their blooms will be blue. In slightly alkaline conditions, on the other hand, their flowers will be pink or even red. Neutral pH can produce purple flowers, or cause a mosaic of pink and blue. Gardeners interested in particular shade of hydrangea can alter the pH of the soil with lime (which alkalizes the soil) or sulfur (which acidifies the soil).
Medicine and agriculture are just two of many fields that make use of the properties of acids and bases. Though it’s easy to relegate the pH scale to a chemistry lab, acids and bases are all around us, and an understanding of how they work comes in handy in more ways than you might expect!
Sources Cited
“Acids, Bases, pH, and Buffers.” Khan Academy, www.khanacademy.org/science/biology/water-acids-and-bases/acids-bases-and-ph/a/acids-bases-ph-and-bufffers. Accessed 1 Sept. 2023.
“Buffers, pH, Acids, and Bases.” Lumen, courses.lumenlearning.com/wm-nmbiology1/chapter/buffers-ph-acids-and-bases/. Accessed 1 Sept. 2023.
Hopkins, Erin, et al. “Physiology, Acid Base Balance.” National Library of Medicine, 12 Sept. 2022, www.ncbi.nlm.nih.gov/books/NBK507807/.
Lesney, Mark S. “A Basic History of Acid -- From Aristotle to Arnold.” Chemistry Chronicles, Today’s Chemist at Work, 2003, pubsapp.acs.org/subscribe/archive/tcaw/12/i03/pdf/303chronicles.pdf.
Oshunsanya, Suarau Odutola. “Introductory Chapter: Relevance of Soil pH to Agriculture.” IntechOpen, 18 Dec. 2018, www.intechopen.com/chapters/64810.
"pH." Encyclopedia Britannica, 3 Jul. 2023, https://www.britannica.com/science/pH.
“pH and Water.” US Geological Survey, US Department of the Interior, 22 Oct. 2019, www.usgs.gov/special-topics/water-science-school/science/ph-and-water.
Kommentare